Course notes and other useful links will be posted here in blog style (reverse order).
Life itself
What can Physics say about life itself? Each living thing is a little self-sustaining non-equilibrium open system. It produces entropy by dissipating energy using the flux of matter and energy that flows through it. The information in the DNA and in the memes, if any, is accumulated by biological and cultural evolution and passed down to many copies of successful organisms. Multicelled organisms are emergent from the cooperation of single cells, each working blindly without central control. The biosphere is a larger version of this game using species. It is ultimately powered by sunlight and connected, via many feedback loops, to the evolution of the whole Earth system. Evolution is not over and, contrary to myth, is as much about cooperation as it is about competition.
There are probably many instances of this whole process operating in our galaxy.
Conway's Game of Life is a startling example of how complexity can emerge from the simple local rules in a "game". You can try it here. The rules applied locally (using only information from nearest neighbours) produce amazing structures globally. Life is deterministic --- there is nothing random about it, except possibly its initial conditions. Life displays Universal Computation and so is, in a sense, infinitely complex. A universal computer, that can in principle calculate or simulate anything that can be computed, has been designed using only Life components. Once a Life pattern is established, it is sensitive to perturbations and has power-law avalanches of activity --- in other words, it displays SOC. If a simple game like Life can display such complexity, what about a soup of organic molecules in some warm little pond?
In this course, we have really just sketched the full scope of emergence in the natual world. Hope you had as much fun as I did.
Tablet notes: [ Lec23 ]
FIN
Self-organized criticality in Politics, Evolution and War
New states emerge when systems are pushed past a critical transition. But certain kinds of systems automatically push themselves to, and remain at, a kind of critical state. The classic example is a sandpile to which sand is being continually added slowly. Eventually, the pile avalanches and the sudden cascades of sand have no intrinsic scale. Their size has a power law distribution. A famous examples are earthquakes which follow the Gutenberg-Richter Law. A simple block and spring model of earthquakes is called the Burridge-Knopoff model. Such systems are said to display self-organized criticality (SOC). This sometimes-overhyped idea is supposed to explain many complex systems from forest fires to the stock market.
Here is a paper about modelling forest fires. Notice it is in a Physics journal! Here is a model of forest fires you can play with. Models like this are ultimately derived from the Ising model of magnetism and are called cellular automata models. The claim is that they display universal behavior that still applies when all the details are included, which is certainly true of the Ising model.
Power laws are often seen (and just as often ignored) in economics, where they signal the possibility of rare but devastating "black swan" events. SOC would seem to imply that such events could be triggered by any tiny everyday ocurrence and that history is always poised on the brink of unpredictable cascading consequences. Lewis Fry Richardson observed that the distibution of the size of wars was a power law long before SOC was invented. Both terrorism and large extinctions in the fossil record have been fitted to power laws. SOC puts us always at the edge of chaos. What are the sociological and political implications of this?
SOC has proved to be a powerful metaphor, at least. But its predictive power seems to be limited. But perhaps this is the inevitable consequence of criticality?
Tablet notes: [ Lec22 ]
Open and closed systems, phase transitions
Collections of identical atoms can behave in macroscopically different ways when their temperature and density are changed. For example, water exists as a gas, a liquid and a solid at different temperatures. Why? These "phases" are collective effects that emerge under equilibrium conditions. The changes, called "phase transitions", are a big object of study in Physics. Changes of temperature in the early universe at which new collective modes of motion emerged, like the symmetry breaking in the standard model, are also usually called phase transitions. Closed systems typically quickly reach thermodynamic equilibrium, after which nothing changes. Equilibrium can usually be assumed if the external conditions on a closed system are changed slowly enough. Open systems, on the other hand, are generally under non-equilibrium conditions.
The distinction between open and closed systems in crucial to the Second Law because open systems do not have to have increasing entropy. The Earth is an open system: it receives energy from the sun and exports an equal amount of energy to the cold night sky. The whole biosphere copiously produces entropy all the time, but this does not mean that the entropy of the biosphere must increase, because it is not open. For a more technical discussion, see here. (Many foolish people believe that biological evolution contradicts the Second Law --- there are many web pages about this -- but this is completely incorrect).
The Ising model of a magnetic phase transition. Here we see how a new ordered structure can spontaneously emerge as some external parameter (here the temperature) is varied continuously. The transition point, often called a critical point, is sharp and the new phase can appear continuously or discontinuously at that "tipping point". At lot has been written about such points, including much nonsense. Near a critical point, fluctuations can become very large as the system becomes "soft" at the transition. Such points are more generally called bifurcation points and they can occur in non-equilibrium systems as well, and can lead to the sudden appearance of new states of motion or even spatial patterns. A good example is the onset of period 2 in the logistic map. Pattern formation is the study of such non-equilibrium structures.
Tablet notes: (Lec 18 -- no notes) [ Lec 19 ] [Lec 20 ][ Lec 21 ][ patterns ]
The arrow of time, irreversibility and the second law of thermodynamics
Why is the future different than the past? What accounts for the persistence of memory? The microscopic laws of physics are time reversible, a fact which is closely associated with the conservation of energy, a deep universal law. Yet we observe a real difference between the past, which stretches back to the Big Bang, and the future which seems as-yet unformed, subject to change and even free will. Why?
Part of the physical explanation of this The Arrow of Time comes from the distinction between macrostates and microstates. Macroscopic systems involve a lot of fundamental particles and have new properties like temperature that individual particles do not. The 19th century science of steam engines, thermodynamics, plus the modern theory of statistical mechanics, worked out by James Clerk Maxwell, J. Willard Gibbs and Ludwig Boltzmann, explains the difference statistically. The motion of complex systems might reverse, but it is extremely unlikely. This is encoded in the Second Law of Thermodynamics, which states that Entropy in a closed system must always increase or stay constant. The three laws of thermodynamics were memorably recited in a song by Flanders and Swan. C. P. Snow also chimed in regarding the Second Law and the Two Cultures.
The Arrow of Time also has cosmological, psychological and quantum mechanical manifestations. But even if we manage to figure out why time has a direction, we are still left with the problem of explaining the Present. The current moment of time, although fleeting, seems different than either the past or the future. This appears to be a problem for which physics has no explanation at all, as it seems to involve the nature of consciousness etc. Consciousness is perhaps the greatest mystery of emergence.
Tablet notes: [ Lec 17 ]
Introduction to chaos
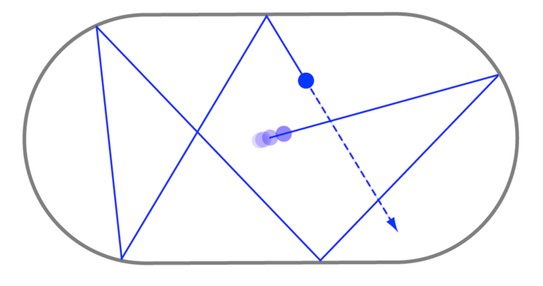
The relationship between determinism and randomness is fuzzier than it might first appear. Even perfectly deterministic classical systems may be chaotic. Chaos was first identified in classic situations like the Three Body Problem, but was not fully appreciated untill computers became widely available in the 1970s.
Chaos is characterized by sensitive dependence on initial conditions, a very common situation in even slightly complex mechanical systems like the double pendulum. This sensitivity is popularly known as the Butterfly Effect, and was made famous by the Jurassic Park movie.
Here is a great overall discussion of chaos. See also the first three chapters from the textbook, Deep Simplicity.
An even simpler situation is that of a Bunimovich Stadium --- an oddly shaped game of frictionless billiards. See a version that shows the sensitivity to initial conditions here. A simplified version of the Three Body Problem can be messed with here.
Ed Lorenz "discovered" chaos while working on a simple model of convection in the atmosphere. It is equivalent to a leaky waterwheel. You can see videos of these here, and here. A more professional discussion is here. Here is an app you can mess with.
Chaos produces effectively random trajectories, even though the underlying Newtonian equations are fully deterministic. Even the tiniest variation of the initial conditions is amplified until it takes over the motion, making long term prediction impossible. A similar problem, only much worse, makes long term weather prediction impossible.
There is a deep connection between chaos and fractals, which will be the next topic of the course. This is exemplified by the chaos game, a random procedure which nevertheless creates a weird sort of order. What emerges is a Sierpinski triangle. Why? Because this shape is an attractor --- the dynamics always approaches it and stays very close to it thereafter.
Physically, chaotic systems necessarily involve nonlinearity. A simple, but not very physical example is the Logistic Map, a sort of crude population model. Here is a site where you can look at the output of this model. Here is a zoomable picture of the bifurcation diagram of this map, which has a remarkable period doubling fractal tree structure.
Lorenz's theory of the atmosphere leads to something called the Lorenz model, whose 3D attractor is can be viewed and messed with here. Ironically, it looks like a butterfly! The three dimensional space that describes the space of this model is called its phase space. The phase spaces of more complex systems, like the double pendulum or the atmosphere, are higher dimensional.
Certainly the most famous fractal is the Mandelbrot set. "Mandel diving" is a geeky way to spend an afternoon. With enough computer power, you can dive very deep. Once you have done that, better eat your fractal vegetables. Or just watch the movie. Phyllotaxis, the process by which plants develop their arrangements of leaves and petals, has close connections with the Fibbonacci numbers and the mysterious Golden ratio. Sometimes, phyllotaxis produces fractal objects, but usually not.
Chaos and fractals seem to represent a new kind of order hiding behind the appearance of randomness. Extended to random fractals, such order becomes quite ubiquitous. Emergent fractal order appears in mundane things like dripping faucets and the shapes of trees.
Tablet notes: [ Lec 14 ] [ Lec 15 ] [ Lec 16 ]
The general features of Emergence
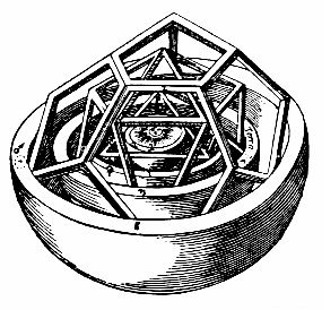
Now that we have sketched the big story of the Universe, let us consider the part played by Emergence in it. Emergence and Reduction go hand in hand in explanation, but Reduction gets all the glory, and Emergence gets all the blame.
One crucial feature of Physical explanation is the separation, introduced by Newton, of Laws and Initial Conditions. Laws can be universal and timeless, while initial conditions introduce messiness and contingency. Another feature of emergence is the role of chance versus determinism. In classical physics, motion is determined, while in quantum mechanics, probabilities are determined, but outcomes are not.
In interaction with symmetry breaking, chance also introduces a large history dependence into Physics. In the standard model, such history dependence is introduced into the deepest features of the Universe, like the masses of the elementary particles, arrived at via the Higgs Mechanism. The Big Bang is the ultimate initial condition. The symmetry-broken Laws are considered to be descended from a more perfect and more symmetric initial state. One book about this idea is even called Perfect Symmetry.
What counts as a Law of Physics anyway? It was once thought that the spacing of the planets was so regular that it had to be accounted for by a law: the Titus-Bode Law. Kepler tried to explain it geometrically. It even works for moons! Now we think it is merely contingent on the initial conditions at the time the solar system formed. How do we decide what needs explanation and what does not?
Are the Laws of Physics really just expressions of purely mathematical truths? Are they as inevitable as 2+2=4? The unreasonable effectiveness of methematics has been commented on. For example, the mathematics of curved space that Einstein used to formulate general relativity had all been worked out previously by Riemann in the 1850s. Was it discovered or invented? This is a perennial question in Philosophy. How can we understand why there are mathematical laws of physics if we can't even understand where math itself comes form? Maybe math is just genetic, something we are wired to do.
What does it mean to "explain" something? What if we can explain a thing "in principle", but not in practice? What if we want to analyze a thing into parts, but, like a proton and its constituent quarks and gluons, it is actually impossible in principle to take the object apart? In the case of such "strong interactions", does it even make sense to talk about parts? Do such things necessarily involve strong emergence? If so, what do we do when reduction fails?
Here are some links to the sand avalanche segregation experiment I showed at the end of class: [ 1 ][ 2 ][ 3 ][ 4 ]. What would it mean to "explain" this experiment?
Tablet notes: [ Lec 13 ]
The hot big bang
It might seem impossible to know anything about the origin of the universe, but Einstein's 1915 General Theory of Relativity, combined with a lot of astronomical observations have turned what was once speculation into quantitative science: cosmology.
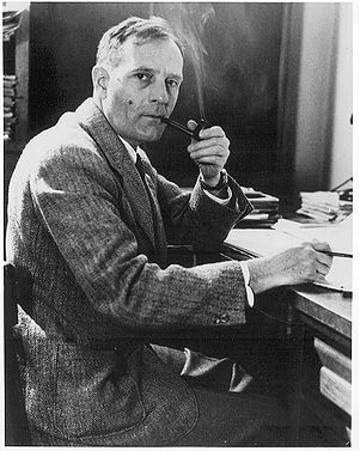
Einstein's theory was first confirmed by Arthur Stanley Eddington's eclipse expedition of 1919. Today many astronomical effects are understood to be due to bending starlight, such as Einstein rings and crosses. See here for more on light bending.
Some of the players in the history of cosmology are: Edwin Hubble, Georges LeMaître and Fred Hoyle. Hubble, who is shown at left, discovered the expansion of the universe and has a famous space telescope named after him.
One of the main tools to understand cosmology is the cosmic microwave background radiation. This is the radiation left over from the Big Bang, now stretched out into a very cold microwave background. It has been measure quite carefully by the WMAP satellite among others.
Here is a map made by WMAP of the deviations from uniformity of the background radiation. These bumps can be used to infer things about the evolution of the universe. A famous cartoon of this evolution is here. The surprizing result is that the universe is composed not only of ordinary matter, but also unseen Dark Matter and even weirder Dark Energy. Here is a pie chart of the composition implied by the observations. Dark energy is a kind of negative pressure that inflates the universe; the discovery of this acceleration won the 2011 Nobel Prize in Physics. Here is a nice news story on all this.
The accelerating expansion of the universe can be incorporated into Einstein's general relativity by adding a "cosmological constant". Einstein originally included this term to fudge his theory into predicting a static universe, a ploy he later called "the greatest blunder of my life".
Working forward from the fluctuations in the density of the early universe, it is possible to simulate the evolution of galaxies, which have an interesting lumpy texture. Galaxies form from the mutual gravitational instability of the matter and dark matter. One can even account for the spiral structure of the Mily Way.
Starting from the standard model and the Big Bang, how do we build a universe? The first stop, just after the symmetry breaking events in the very early universe, is when the quarks assemble themselves into baryons (protons and neutrons). The universe is then a hot plasma of ionized hydrogen, which eventually cools and becomes transparent. At this stage the background radiation escapes. Then this neutral cloud collapses into stars and galaxies and other stuff like early black holes and quasars. Along the way, all the elements heavier than Hydrogen are synthesized first by a little cooking just after the big bang, and then later in the interior of stars.
Nuclear fusion joins protons and neutrons into heavier nuclei. Stellar evolution returns the matter back to the interstellar medium, which can reform into new stars for more cooking. If they are cold enough, nuclei collect electrons to become atoms. Even the same element can have different arrangements of protons and neutrons in the nuclei. The elements can be combined into a famous song. Which is sometimes sung by famous people. Then atoms of the various elements to may be linked by chemical bonds to form molecules, and eventually important biomolecules.
Atoms are something we can "see" directly, for example using a scanning probe microscope. Once can even move individual atoms around using such a microscope. Normally, objects the size and weight of atoms and molecules behave as classical physics would predict, so the chemical bond is approximately the limiting size at which quantum effects are important in everyday Nature. Of course, there are famous exceptions like superconductors (see video) or superfluids (see video).
Tablet notes: [Lec 11] [no notes for Lec 12 ]
The particle zoo: climbing the chain
The standard model of particle physics forms the lowest level building blocks that we know. It contains three of the four fundamental forces (electromagnetic, and the strong and weak nuclear forces); only gravity is left out. These forces are created by the exchange of particles. All these particles fit on the T shirt.
How did the fundamental particles get these particular properties? Why do we have the elements that we do? The explanation for these facts lies in the early universe, and hence involves cosmology and Einstein's general theory of relativity. The explanation involves the idea of spontaneous symmetry breaking shortly after the Big Bang.
The crucial idea is spontaneous symmetry breaking. In the beginning, everything is massless and simple and the properties of the elementary particles come from "breaking" this highly symmetric situation as the universe expands. One obvious case, which is not really understood yet, is the breaking of the matter/antimater symmetry. Another is the Higgs Mechanism -- which involves a new particle that is supposed to explain the origin of mass. See here for a nice story on how this cocktail party analogy came to be. The Higgs boson is one of the main things being looked for at the LHC. The latest info on the search at ATLAS is here. More on the Higgs mechanism here.
Here is a little cartoon of how things are supposed to have fallen out after the Big Bang.
Tablet notes: [Lec 8] [Lec 8.5] [Lec 9][Lec 10].
Forces and Particles
Electric and magnetic forces were added to Newton's picture in the 19th century, most famously by James Clerk Maxwell (shown here). Later, the last two nuclear forces, the "strong" and "weak" nuclear forces came along to explain very small phenomena. The whole modern picture of forces consists of Einstein's general relativity, which dominates explanations on the largest scales, and the quantum theory plus special relativity, combined into the standard model of particle physics, whiich is applied to the smallest scales. It all fits on a T shirt. But gravity is not integrated properly yet.
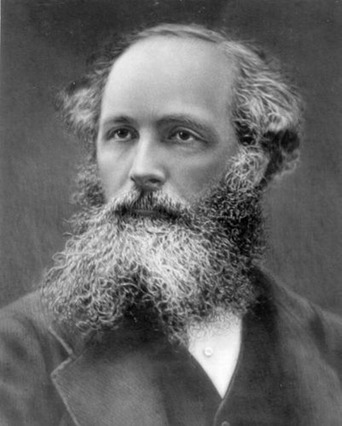
One way to organize the chain is to ask which of the basic forces dominates at each scale. Then the various explanations have to be fitted together, and this is sometimes difficult. The standard model does not include gravitation. The transition from the quantum world to the classical (Newtonian) world is notoriously problematic.
Much of what we know about the nucleus and the fundamental particles comes from scattering experiments. The grandaddy of all scattering experiments is the Rutherford Scattering Experiment. Studies of cosmic rays were also important, before particle accelerators took over.
Today, giant accelerators like the Large Hadron Collider (LHC) at CERN create powerful beams of particles which are used to probe down to scales much smaller than protons. Huge detectors like the ATLAS detector at the LHC are required to measure all the particles that emerge from collisions. Here is a good diagram of the detector. You can watch the data arrive live here. The Standard Model of Particle Physics describes the dynamics of the various types of elementary particles which inlcude leptons (like the electron) and hadrons (like the proton and neutron, which are composed of quarks). Hadrons are further classified into mesons and baryons: 2 quarks for a meson, and 3 for a baryon.
List of mesons, List of baryons. Most of these particles are unstable and decay into simpler stable particles. All particles made of quarks are collectively called hadrons.
Recently, an experiment involving neutrinos made the news because it appeared that the neutrinos had been caught travelling faster than light. This result would be earth shattering, if it is true. You can read some nice news stories about it here, and here, and many other places. You can have a go at reading the original scientific paper on the experiment here. Needless to say, people are quite skeptical about the correctness of this experiment and it will certainly be closely tested before being accepted.
Tablet notes for lecture 5 here. Lecture 7, here.
The modern "great chain of being" and emergence
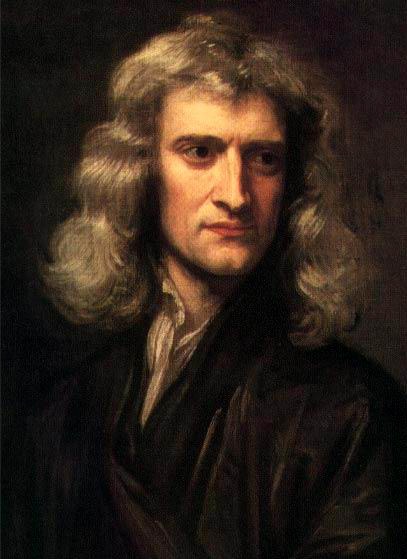
The modern great chain of being links scales from the size of the universe to the size of the most findamental particles we know. Explanation in terms of smaller simpler parts is the standard reductionistic method of science. Going backward, up the chain, involves emergence. "Weak emergence" is the claim that everything is reducible in principle, even non-obvious properties of complex systems --- but that such reduction is merely impractical. "Strong emergence" is the claim that some properties are irreducible --- that sometimes the whole is different than the parts, even in principle. This idea is much less accepted scientifically.
How was this chain assembled? The idea of atoms --- as ultimate unbreakable particles --- goes back to the ancient greeks, like Democritus. The roman poet Lucretius gives an account of the universe in terms of differently shaped atoms. But atoms actually came along much later via Chemistry. They were not really incorporated into Physics until the quantum revolution in the 1920s.
The real Physics synthesis behind the chain begins properly with Isaac Newton. He formulated the first "universal" laws and emphasized the idea of forces. His laws of mechanics and gravitation stood until Einstein modified them in the early 20th century. Most famously, Newton showed that his law of gravity resulted in orbits that are conic sections. Conic sections are a staple of ancient greek mathematics, once again showing the "unreasonable effectiveness of mathematics".
Class notes from the tablet are posted here. And here.