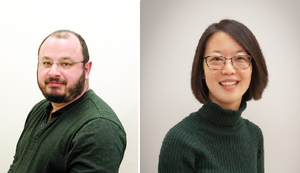
"Self-regulation of the nuclear pore complex enables clogging-free crowded transport” published in February 2023 in Proceedings of the National Academy of Sciences (PNAS)
Authors: Anton Zilman and Tiantian Zheng (left to right)
In all eukaryotic (that is non-bacterial) living cells -- from yeast to humans -- the carrier of genetic information, the DNA, is sequestered in a separate compartment -- the cell nucleus. This ancient arrangement endows our cells with many benefits such as more complex regulation, resistance to mutations, and evolvability. However, it also poses a difficult transport logistics problem as the separation of the nucleus and the rest of the cells necessitates large-volume -- millions of molecules per second -- molecular transport between the two compartments.
All this enormous transport is facilitated by biological "nanomachines" known as the nuclear pore complexes (NPCs). NPCs are one of the most complex and largest molecular assemblies in the cell, comprising dozens of different molecule types in large copy numbers. Yet, surprisingly, our previous work has shown that despite this complexity, the molecular architecture and the transport mechanism of the NPC can be understood based on relatively simple physical principles. Partially due to this, NPC has served as an inspiration for creation of artificial nanopores for molecular sorting for bio-nano-technology applications.
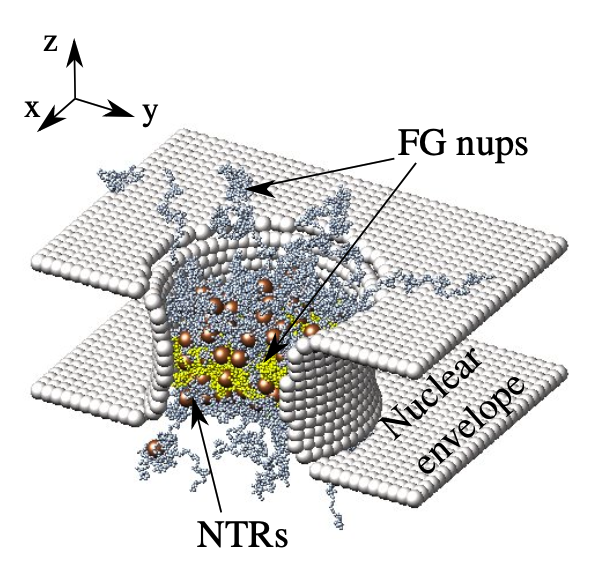
This figure provides a snapshot of a minimal complexity simulation of the Nuclear Pore Complex (NPC).
In this particular work, we have a developed a physical model of the NPC to investigate one of the puzzles of its function -- how can it maintain rapid, efficient, and selective high throughput transport despite the fact that its transport channel is highly crowded with many simultaneously translocating cargo-carrying transport proteins? Past experimental results suggest that the NPC is surprisingly resistant to clogging and that transport may even become faster and more efficient as the crowding in the channel increases. Our model explains these puzzling observations and shows how the unique internal architecture of the NPC engenders clogging-relieving mechanisms that—counterintuitively—harness crowding toward faster and more efficient transport. These results explain long-standing puzzles and suggest design principles for the optimization of artificial selective nanochannels inspired by the NPC.
Interview with the authors of this research:
What is the relevance of looking at transport systems in cells as a physicist?
As physicists, we are interested in understanding the world we live in using the tools of mathematics. While biological systems present many challenges through their sheer complexity, non-linearity, non-equilibrium nature, and stochasticity they are governed by the laws of Physics as much as any other system in the universe. Understanding how cellular structures function and are regulated is a key aspect of identifying how physics produces the myriad of diverse phenomena that characterize life. Specifically, we are using the tools of non-equilibrium statistical physics to understand transport phenomena on the nanoscale by various biological "nanomachines".
Is this research used to diagnose or treat medical conditions or contribute to medical techniques? Please share if this is part of a current medical practice.
The particular "transport machine" of interest for us -- the nuclear pore complex (NPC) -- is implicated in diseases such as cancers and neurodegenerative diseases, and one mechanism for disease is the clogging of the NPC by amyloids. However, during healthy cell function, the interior of the NPC is also extremely crowded with a multitude of different cargoes being transported, which apparently doesn't lead to clogging. Our research set out to understand how crowding is managed by the healthy cell, and how it prevents normal levels of crowding from escalating into clogging. So yes, our research builds the foundation for a better understanding of the dysfunction of the cellular transport in pathological conditions, and development of better treatments.
What is the long-term impact of this research?
By understanding how the NPC operates normally, we hope that in the long term this will allow us to identify abnormal conditions, and how to correct them therapeutically. The operation of the NPC also has served as an inspiration for creation of artificial nanochannels for various bio-nano-technological devices such as protein sorting and detection with applications ranging from disease detection to water purification. On the mode basic side, our work has provided new theoretical and computational tools to deal with complex biomolecules in confined spaces on the nanoscale.
What challenges do you face in this field of research and how do you overcome some of these challenges?
One of the main challenges in this field of research is the difficulty of experimentally probing the NPC directly in live cells. Our methods of physical modelling strongly depend on having high-quality experimental data with which to compare to or fine tune our models on. However, the small size of the NPC (~10 nm), and fast time-scales of the dynamics of NPC components make direct experimental characterization of the NPC difficult. Studies measuring transport properties are typically conducted in cells, and such experiments are extremely resource and time intensive. In our work, we have relied on synthesis of multiple streams of data -- from in vitro experiments on the biophysics of the NPC components to single molecule tracking in live cells -- to identifying the "consensus" features between different results from different labs to inform our modelling approaches. Another big challenge in an inter-disciplinary field is to bridge the gaps in scientific cultures and ways of thinking about the problem between very disparate fields, including theoretical physics, cell biology and biochemistry, and the success of our research really derives in a large part from long-standing collaborations with experimentalists in different fields.
What is your area of research and why did you choose to specialize in this field?
TZ: I have been working on research in biophysics since my third year of undergraduate studies. I was drawn to the field because of the variety of interesting problems that touched on some of my favourite areas of physics (in particular stochastic processes and nonlinear dynamics)
AZ: I was always attracted to inter-disciplinary problems (one discipline is too boring!). My PhD is in statistical physics/physical chemistry, and I was drawn by the challenge of understanding principles of function of very complex non-equilibrium systems that are present in living organisms using relatively simple physical and mathematical models.
What else are you working on?
TZ: We have just recently finished a follow-up paper which addresses how the NPC avoids clogging when cargoes are transported through the pore in both directions at the same time (whereas the highlighted paper focusses on transport in one direction). We again found several novel regulation mechanisms which arise through the collective dynamics of the system, some of which could potentially be applied to increase the performance of artificial nanoscale filters which are inspired by the NPC.
AZ: Beyond cellular transport, my group is generally interested in how various biological processes can be so precise and specific despite ubiquitous noise on molecular and cellular scales, including molecular signaling and spatial organization of multi-cellular collectives. One area in which we are very active now is signaling in the immune system, trying to understand the molecular signaling "code" using tools of statistical inference theory and machine learning.
Read the full paper here: https://www.pnas.org/doi/abs/10.1073/pnas.2212874120
More on Professor Anton Zilman’s Group here: https://www.physics.utoronto.ca/~zilmana/